An Overview of Geothermal Resources
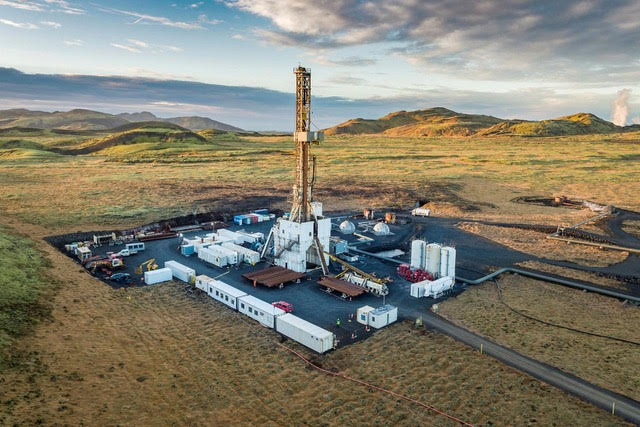
Picture: Iceland Drilling rig Thor on site in Hverahlid, Iceland (source: Iceland Drilling)
Geothermal energy can be harnessed for the use of its heat directly (direct use) or for electricity generation. Geothermal energy is thermal energy which is generated and stored within the Earth, as we described in our previous chapter on What is Geothermal Energy?
In the context of how one derives this energy we define four categories of geothermal resources:
- Shallow geothermal heat-pump, or geo-exchange resources
- Hydrothermal resources
- Enhanced/ engineered geothermal systems
- Unconventional or advanced geothermal systems
The type of resource determines how we can extract the thermal energy from the ground for extraction for energy utilisation on the surface.
[Picture: The sources of geothermal energy – a graphic by Green Rock Energy, Australia ca. 2009 – the picture is missing “Advanced Geothermal Systems“]
1. Hydrothermal Resources
Hydrothermal, refers to heated water resources, which can be found in hydrothermal resources that are naturally occuring. They are created by groundwater and favourable rock characteristics, such as open fractures or fissures, that allow the flow of fluids between.
With the high(er) temperatures of the rocks, the fluids are heated and can be derived either as hot water or steam, if the temperature is high enough. So the fluid, or steam carry the heat which can then be used on the surface for either heat applications, or for electricity generation.
These hydrothermal resources range in temperature from a few degrees above the ambient conditions on the surface to temperatures beyond 350 degrees Celsius (or 660 Fahrenheit).
Hydrothermal resources can be found in volcanic settings (such as in Indonesia), in sedimentary settings (such as the German Molasse Basin) and hot wet rocks (e.g. fractured granite with water resources).
2. Unconventional Resources
Unconventional resources, such as either enhanced or engineered geothermal systems, or new so-called advanced geothermal systems, are approaching geothermal resources (heat) that lack the necessary fluids or rock characteristics that would allow for heat extraction.
Unconventional resources are geothermal energy that can be found in hot dry rocks for example – essentially hot baserock settings, where there is heat, yet no water flows that could be extracted as a carrier of the heat.
So, one would require some element of heat exchange to extract the heat from the subsurface for energy utilisation above the surface, either for heat or power generation.
i) Enhanced/ engineered geothermal systems (EGS)
The approach in enhanced geothermal systems (EGS) is to create permeability between rocks and adding fluids that would allow them to be sufficiently heated in a sustainable system (and thereby artificially create hydrothermal ‘reservoirs’).
This allows one to derive geothermal heat from beneath the surface to be used on the surface. As one creates these reservoirs artificially, one often also calls them engineered geothermal systems.
Another term used often is Hard Dry Rock (HDR). In the past the term was used a lot in the Australian context, whereby ‘hard’ described the non-permeability of the rock and ‘dry’ described the fact that there were no fluids that would have allowed the extraction of heat in the traditional or conventional way.
With traditional hydrothermal resources often tapping higher temperatures in accessible depths, (e.g. along the tectonic plates) EGS systems allow the tapping of geothermal energy beyond these areas essentially around the globe.
Geothermal energy can be found around the globe, yet the level of depth, the temperature and the availability of fluids have determined development so far.
The challenge with EGS technology is the cost involved in targeting sufficient temperatures in depths that are often much higher, and the economics of creating a sustainable system that would allow utilisation for the long-term.
Drilling costs, stimulation of rocks to make them permeable, the pumping of water both down into the artificial ‘reservoir’ and its pumping to the surface requires in itself a lot of energy. The temperatures are also often not as high, hence the technology to generate electricity is also more complex and more expensive.
The element of ‘stimulation’ is also often seen as critical as the pressure applied can create small earthquakes on the surface that – particularly in urban areas – create concerns of the public and other stakeholders.
While EGS systems are often described as separate systems, EGS technology can also be applied in conventional geothermal settings.
Stimulation can help make hydrothermal reservoirs more productive by increasing permeability and thereby increase output on the surface.
[Picture: Diagram showing the production of electricity using enhanced (engineered) geothermal systems, picture source: U.S. Department of Energy]
Today, there are a large number of examples, where EGS techniques have been applied to increase performance of hydrothermal resources, and a number of EGS have been developed (e.g. in France and Australia). But today, there is – to the best of our knowledge – no pure EGS system that produces electricity today.
EGS systems can also provide heat in a direct use context (e.g. for district heating networks and other industrial heat applications).
The potential for EGS in the United States alone is estimated at around 5,150 GW, according to the U.S. DOE Geovision Report of 2019 referring to Augustine 2016, and Augustine et al. 2019.
To put this in context, this would represent five times the total installed utility-scale power generation capacity in the U.S. as of 2016.
(ii) Advanced Geothermal Systems
The concept of advanced geothermal systems (AGS) has been coined by various groups targeting an approach that would remove the resource risk in geothermal development, namely the need to find sufficient temperature, find sufficient fluids, or in the case of EGS create sufficient permeability.
AGS does so by extracting thermal energy utilising a closed-loop system. It achieves this by circulating a working fluid through a long wellbore that conducts heat from the rock surrounding the well.
These concepts have been discussed in science for a long time, either as a single well solution, or with new efforts gaining a lot of attention in a closed loop system, not quite dissimilar to shallow heat exchange systems.
These closed-loop systems are built on wells drilled that connect with each other allowing a heat exchange-type set up beneath the surface – at deeper levels than traditional geo heat-exchange systems.
These systems would allow the extraction of geothermal heat virtually everywhere on the planet. Pilot projects have proven the concept of these closed-loop systems. The planned development of commercial scale systems will show how the economics will make these systems a valid and competitive option for both power generation and direct use.
[Picture: The conceptual model of a closed-loop heat exchange through connecting wells in hot rock settings to extract the heat, here the concept of the pilot project by Eavor Technologies, Inc. From Canada – picture source: Eavor Technologies]
There is yet another new approach to utilise geothermal energy which is the utilisation of so-called supercritical geothermal systems. If they would fall under the category of ‘Advanced geothermal systems’ is likely a bit questionable, yet it is also an advanced approach to tapping geothermal energy.
The concept of tapping supercritical geothermal systems is tapping super high temperatures of 400 degrees Celsius (750 degrees Fahrenheit).
The idea is that the energy content is so much higher that it would make the energy output per well much higher than in traditional systems.
This reservoir fluid is assumed to be in a ‘supercritical’ state, as fluid, water and pressure are so much higher.
With temperatures in excess of 370 degrees Celsius and a pressure of 220 bars and more, these wells could be much more powerful.
The Iceland Deep Drilling Project (IDDP) is likely the most prominent project looking at exploring how one can tap this powerful energy. While a traditional geothermal well in Iceland produces maybe around 5 MW, a well with supercritical conditions could potentially produce ten times that amount.
The management of such high temperature resources, high pressure and often the composition of the brine fluid are presenting a challenge. A lot of research remains to see how an approach to expand geothermal energy extraction from supercritical fluids will have to be seen.
[Picture: Conceptual diagram of wells for geothermal energy production from supercritical fluid. Credit: By G.Ó. Friðleifsson, The Iceland Deep Drilling Project | National Energy Authority of Iceland]
Geothermal Heat Pumps
Geothermal heat pumps are also often referred to GeoExchange, ground-source or water-source heat pumps.
In the geothermal sector there is therefore also a distinction made between deep and shallow geothermal energy extraction.
The main concept can be described as the utilisation of the constant temperature of the earth as an exchange medium instead of the temperature outside.
With wider application of heat exchange systems and deeper depths sought in the context of geothermal energy extraction, the lines between what constitutes deep and shallow geothermal therefore becomes more difficult to determine.
For our purpose, we are also only looking at heat pumps utilising the energy of the earth, not air based heat exchange systems.
The geothermal cooling and heating system essentially has three main components:
- The Heat Pump
- The Heat Exchanger (a closed-loop system)
- The Distribution System (distributing the heat or cooling in a house)
The ‘pump’ uses electricity to derive the fluid from the loop system (either vertical, horizontal, or even from a pond/ lake) using a liquid heat-exchange medium (water or refrigerant based) and the delivery system, which can be an air-delivery system or radiant heating.
The fluid is circulated through the loop pipes buried beneath the surface, or – if water based – through lakes, or mines.
The general concept is that the ‘pump’ then derives heat from the ground in the winter time for heating, while also being able to get the heat out of buildings to cool in the summertime, as a heat sink. So, essentially the heat pump transport heats.
The heat pump “functions in the same way as a compression refrigeration system, except that the principal focus is not on the evaporator side, i.e. the cool side, but on the condenser side where the heating effect is. The essential principle remains the same: evaporation of refrigerant in the evaporator, increase of pressure and hence temperature in the compressor, dissipation of the gained heat by liquefying the refrigerant in the condenser, and subsequent expansion of the refrigerant through the throttle valve. The refrigerants used are also hardly any different from those used in an ordinary refrigeration plant.“ (source: Danfoss)
The efficiency of these heat pumps are described based on their coefficient of performance, or COP. COP is a scientific way of determining how much energy a system ‘generates’ versus how much it ‘requires’ to operate.
Utilising the pump, which uses electricity, the fluid of the loop system then extracts temperatures of around 7 degrees Celsius or more from the ground (or water), and pushes it through the heat exchange system that in a way works like a refrigerator generating heat, or in the summer cold.
Most common heat pumps of COPs of 3.0 to 5.0. This means that for each unit of energy used to power the system (e.g. 1 kWh of electricity), it creates 3.0 to 5.0 KWh of thermal energy.
These systems can also extract heat from subway tunnels, industrial waste heat, or abandoned coal mines.
[Picture: Geothermal heat pumps and closed-loop elements, picture source: University of Boras, https://techxplore.com/news/2020-11-talc-pipe-geothermal.html]
Direct Use of Geothermal Energy Resources
The ‘direct use of geothermal energy resources’ describes the direct use of the heat, rather than generating electricity from heat indirectly – e.g. through steam from geothermal resources which turns a turbine to generate electricity.
For the generation of electricity, temperatures need to be sufficiently high to create steam that can turn a turbine, yet there are a lot of applications that utilise heat.
The most obvious is naturally space heating or heating water for swimming pools. But there are much wider applications, such as for food processing, and heating greenhouses etc.
One of the pioneers of direct utilisation of geothermal energy was Baldur Lindal (1918-1997).
Baldur Lindal was an Icelandic chemical engineer who worked for more than five decades in geothermal research in Iceland and internationally. His focus was on the development of processes for direct applications of geothermal energy for industrial purposes.
In a research paper in 1973, he created a diagram to show what temperature resources could be used for what purposes. The ‘Lindal Diagram’ has become the main showcase for direct use applications that can utilise the heat of geothermal energy directly, hence the term, ‘direct use’.
[Picture: The Lindal Diagram, after Lindal 1973, Geothermal Education Office 2005, picture source: https://www.researchgate.net/figure/The-Lindal-Diagram-after-Lindal-1973-Geothermal-Education-Office-2005_fig17_317388065]
The best and most visible example of utilising geothermal energy in the direct use sense are the natural hot springs of this world.
Bathing in the comfort of hot water is the most obvious and relatable example, yet there is naturally more, such as growing vegetables in greenhouses year-round in colder climates.
[Picture: Blue Lagoon with Svartsengi plant, Iceland (Picture: Vestman, (c) CC BY 2.0)]
[Picture: ORF Genetics greenhouse in the winter landscape of Iceland (source: ThinkGeoEnergy)]